Raphael Horvath1, Max Stammet1, Florian Eigenmann1
1) IRsweep AG, Laubisrütistr. 44, 8712 Stäfa, Switzerland
Although many chemists and chemical engineers work in the solution phase, a vast number of reactions occurring in nature and in industry happen between phases, that is, they are heterogeneous. For catalytic reactions, if the catalyst is in a different phase to the reagent, the process is known as heterogeneous catalysis. This application note shows that it is possible to integrate commercially available diffuse reflectance accessories with the IRis-F1, to achieve diffuse reflectance measurements on the microsecond timescale.
Doing so combines the high optical power of the IRis-F1 with the light hungry but powerful technique of diffuse reflectance. This gives sensitivities and time resolutions not achievable with other commercial mid-infrared spectrometers.
Experimental setup
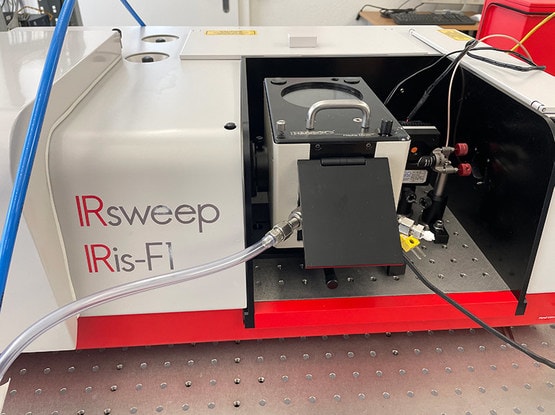
We coupled the IRis-F1 to a Praying Mantis diffuse reflectance accessory (Harrick Scientific), as shown in Figures 1 and 2, below. The solution works “out-of-the-box”, although further optimization can improve the throughput (see below). An external MCT detector is used to collect as much light as possible.
In this note we show a photophysics applications and a gas flowing application. For the first, the dye Erythrosin B mixed with sucrose was used, since we expect this to possess an excited state lifetime of 100s of microseconds, based on previous studies in the UV-Visible spectral region. To our knowledge, no excited state infrared spectrum of this compound has been published. Erythrosin B in a 1:10 ratio with sucrose was placed in the open sample cup in the Praying Mantis accessory. Second harmonic 532 nm radiation (2 mJ/pulse) from a Nd:YAG laser (Spitlight II, Innolas) was used to photoexcite the sample.
The infrared signal was recorded for 2 ms for each pump pulse and each pulse is timed to arrive halfway through the acquisition window by triggering the IRis-F1 from the Q-switch output of the Nd:YAG laser. This way, the first 1 ms of the acquisition is used as the background to calculate the sample reflectance. The experiment was repeated 500 times to improve the signal to noise ratio, giving a total experimental time (including data processing) of ca. 90 seconds.
For the second experiment a gaseous species flowing into the high-temperature reaction chamber of the Praying Mantis was observed. A titanium dioxide surface is monitored while a flow of acetone is introduced on a stream of nitrogen carrier gas. Here, the IRis-F1 is set to acquire with a frequency of 10 Hz and a background measurement is taken before the experiment.
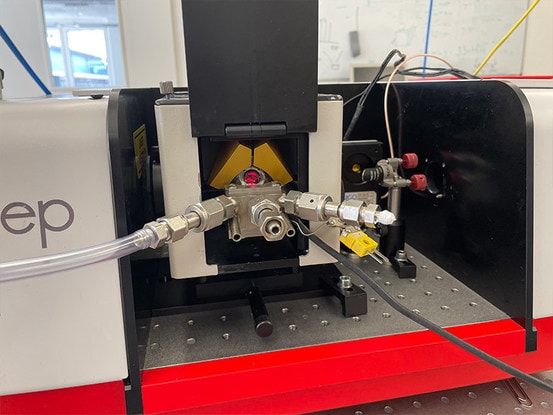
Results and discussion
Time resolved infrared spectroscopy of Erythrosin B
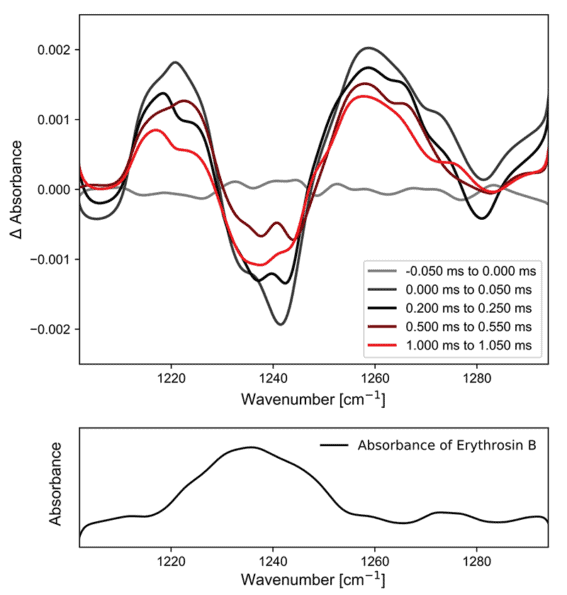
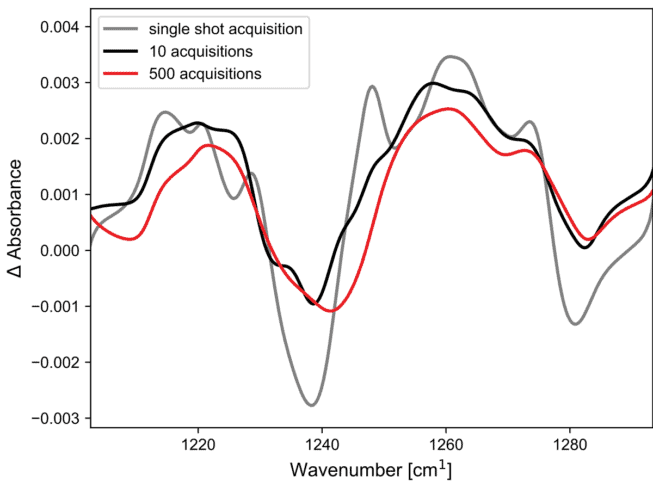
The top panel of Figure 3 shows the time resolved infrared spectra of Erythrosin B after photoexcitation with 532 nm light, measured in diffuse reflectance. The bottom panel shows the ground state spectrum of the same sample, taken against a KCl background.
In the representation of Figure 3, negative spectral features correspond to species that have disappeared while positive features are due to new species that have been generated. Immediately after photoexcitation the ground state band is bleached, indicating that a new state has been formed. This new state has infrared bands at approximately 1220 cm-1 and 1260 cm-1. Over the course of the next millisecond, these bands diminish while the ground state recovers. The kinetics of this process can be fit to give an excited state lifetime of approximately 460 µs, which is consistent with the previous investigation.
To understand the signal level achievable by single or few-shot experiments, the averages of 1, 10, and 500 acquisitions were plotted, where each trace is integrated over 0.5 ms. As show in Figure 4, the spectral features are very well reproduced with 10 acquisitions, and even with a single shot experiment, usable data are generated. This shows that single-shot time-resolved diffuse reflectance spectroscopy is a possibility with the IRis-F1.
Gas phase spectroscopy in the high temperature reaction vessel
Acetone was pumped into the high temperature reaction vessel on a stream of nitrogen carrier gas and at the same time, the infrared reflectance of a sample of titanium dioxide was measured. The results are shown in Figure 5. The asymmetric C-C stretching bands of acetone at 1218 and 1229 cm-1 are seen to grow over a timescale of seconds. In this initial experiment we are likely observing the acetone headspace over the top of the catalyst, however it nicely demonstrates that gas phase species can be measured in the Praying Mantis cell.
These experiments form the basis of photocatalysis or gas-flow experiments that can be carried out with such a setup in real-time.
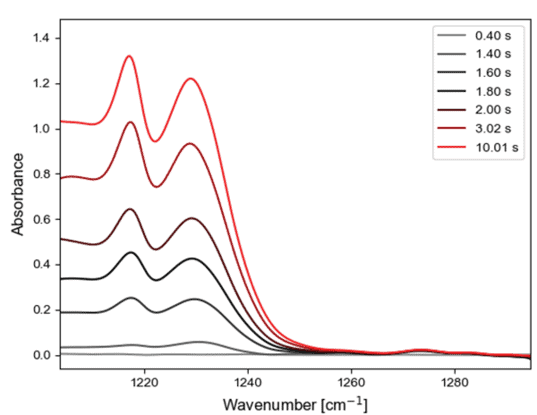
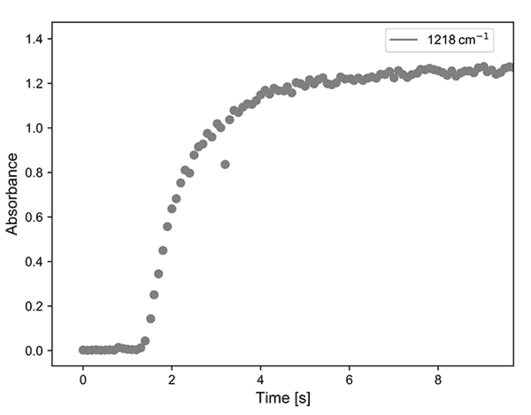
Figure 5. (left) Infrared absorption of acetone as it is pumped into the Praying Mantis cell; (right) kinetic trace of this process.
Note on compatibility
Good “out-of-the-box” compatibility was found between the Harrick Praying Mantis cell and the IRis-F1. Although the Praying Mantis is designed for use with a focused beam, as is found in an FTIR spectrometer, a good focus was achieved on the sample. On the exit port of the cell, mounting the detector next to the Praying Mantis is recommended, since the output beam is divergent. The standard optics used in the IRis-F1 can be used to mount the detector to give good light throughput.
Conclusions
The Praying Mantis is an excellent tool for studying solid materials for use in heterogeneous catalysis. We have found good compatibility with the IRis-F1, and this allows us, for the first time, to observe heterogeneous and solid-state reactions with a microsecond time resolution. This is a light-hungry application, particularly with weakly scattering samples. Combining diffuse reflectance with a laser based spectrometer opens the door to many new investigations and working with faster reactions with minimal experimental overhead.