Raphael Horvath1, Jeff Deischter2, Maurice Vennewald2 and Regina Palkovits2
1) IRsweep AG, Laubisrütistr. 44, 8712 Stäfa, Switzerland
2) Institut für Technische und Makromolekulare Chemie (ITMC), RWTH Aachen, Germany
Heterogeneous catalysis is an extremely important field of research, underpinning many industrial processes. It is defined by catalysts that are in a different phase to the reaction materials with which they interact, for example, a solid catalyst interacting with liquid or gaseous reagents. This is attractive from a processing perspective because catalysts and products can easily be separated. It also means that reactions typically occur on surfaces or interfaces.
Studying the surfaces of materials is an important step in understanding and influencing heterogeneously catalyzed reactions.
A powerful method to obtain optical spectra is diffuse reflectance spectroscopy, which makes it possible to obtain absorption spectra from non-transmissive samples and has been widely applied from the UV to the infrared. As implied by the name, light is diffusely reflected from a surface before being collected (see picture below). Since it is a very photon-hungry technique, to achieve a good signal to noise ratio it is important to collect as much light as possible.
Since the IRis-F1 is based around high power frequency comb lasers, it can be an ideal tool to study solid samples in diffuse reflectance, including in challenging conditions, such as for time-resolved measurements. This application note shows how even a simple optical setup can be used to obtain high-quality diffuse reflectance spectra of dark samples.
Experimental setup
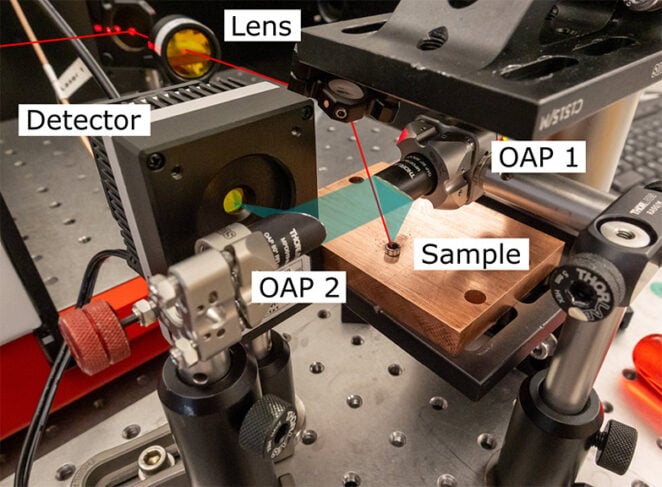
The experimental setup aimed for simplicity, to understand whether the IRis-F1 could be used for diffuse reflectance applications. The geometry of the sample setup is shown in the picture.
The incoming beam (red) arrives at the sample from the top and is focused by a f = 100 mm lens. It has a power of approximately 30 mW. Light is collected (blue) at a diffuse scattering angle with an off axis parabolic mirror (OAP 1) and subsequently focused on the detector with a second mirror (OAP 2). The powdered sample is held in a steel disk to achieve a reproducible surface height.
Samples
A series of carbon carbon samples were kindly provided by Jeff Deischter from the Palkovits group at RWTH Aachen. These were activated coal, oxidized activated coal, and both of the above with l-arginine adsorbed on their surfaces. Such carbon materials were previously found to have a very low reflectivity, and are therefore challenging targets for diffuse reflectance spectroscopy. Indeed, we measured a reflectivity of approximately 0.1% in this configuration, which collects about 1/8th of the light scattered over a hemisphere.The aim of the investigation was two-fold: firstly, can we distinguish between the oxidized and unoxidized carbon samples, and secondly, can we detect the presence of l-arginine on the surface of these samples. As depicted, arginine is expected to show a C=N stretch around 1630 cm-1.
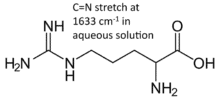
Data acquisition and analysis
For each measurement a background was acquired on an appropriate sample without an analyte. In the case of the carbon samples, we chose KBr to provide the background. In the case of arginine deposited on carbon, we chose the respective bare carbon sample.
To make the measurements comparable to one another, the reflectivity was converted to the Kubelka-Munk scattering parameter, according to the following equation:
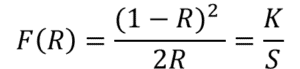
Where R is the reflectivity, K is proportional to the extinction coefficient and S is the scattering coefficient. This means positive features in the spectrum correspond to absorption by the sample.
For each measurement, a total integration time of ca. 400 ms was used and spectra were averaged to a resolution of ca. 4.5 cm-1.
Results
The first measurement was of the two samples of carbon against a KBr background. Broad features are seen for both samples, rising at lower wavenumbers. An important difference is a band at the high-wavenumber end of the spectrum of the oxidized sample. The is consistent with the presence of possible C=O species on the surface of the material, which are expected to occur in the 1700 cm-1 region of the spectrum.
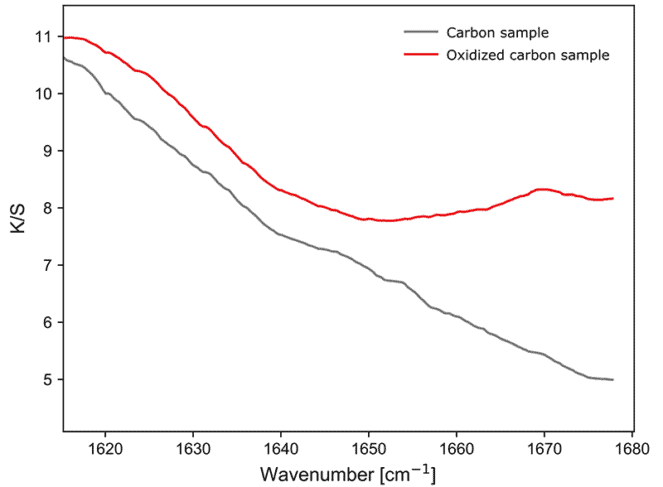
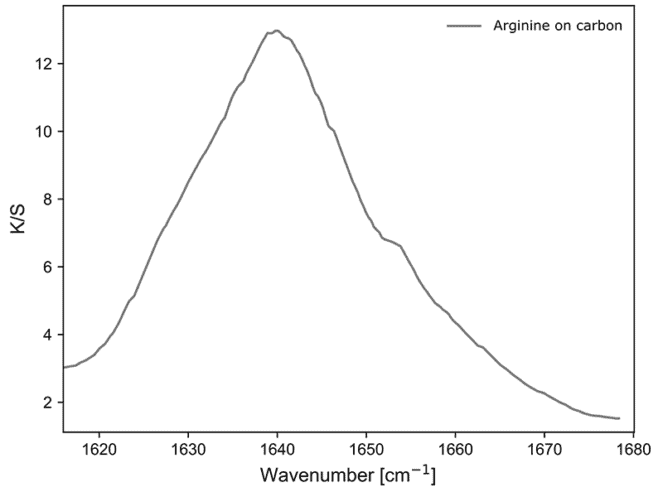
Next, l-arginine on the carbon surfaces was investigated. To prepare, we initially recorded a reference spectrum of solid l-arginine against a KBr background. As expected, we observe a peak around 1640 cm-1.
On the carbon samples, the bands of arginine are more subtle but still visible. On the standard carbon sample the band appears weak and slightly shifted to lower wavenumber values. On the oxidized carbon, the band is shifted to slightly higher wavenumber values. This shows that it is possible to investigate the modification of the C=N bond of l-arginine while bound to different samples of highly absorbing carbon, even at short integration times.
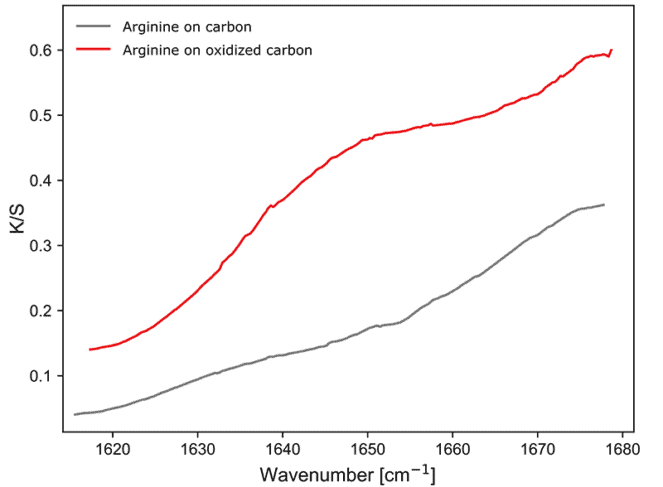
Conclusions and outlook
These experiments have shown that it is possible to obtain good spectra in a diffuse reflectance configuration, even with strongly attenuating samples. Using a relatively simple setup, changes in the spectrum of l-argininine upon biding to different forms of carbon could be measured repeatably.
Future experiments will focus on time resolved measurements. Currently an integration time of ca. 400 ms is used, but this can be shortened if needed. By using collection optics with a higher numerical aperture, and more precise alignment, it will be possible to collect considerably more light and we expect achievable useful time resolutions in the low milliseconds for future experiments. This opens the door to measuring fast time resolved changes on surfaces in the mid-infrared, providing a valuable tool for the investigation of hetereogeneous catalysts in action.
Acknowledgement
These experiments were made possible thanks to samples provided by Jeff Deischter from the research group of Regina Palkovits at the Institut für Technische und Makromolekulare Chemie (ITMC) in Aachen.